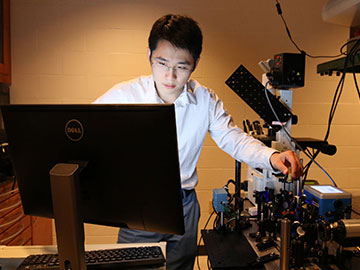
Purdue postdoc Delong Zhang is lead author on a study that unveils a new mid-infrared photothermal microscope technology. The approach uses a thermal-lensing effect from a mid-infrared laser to allow high-resolution chemical imaging of living tissues with a second, visible-light laser. [Image: Purdue University/Erin Easterling]
Driven especially by advances in quantum cascade lasers (QCLs), infrared (IR) absorption spectroscopy has earned its stripes in recent years as a label-free technique for imaging a wide range of materials. But for live biological samples, the mid-IR band has some notable drawbacks. Those shortfalls include spatial resolution on the order of 4 to 7 μm, far too coarse to pick up intracellular structures, and strong absorption by water, which has limited use of IR spectroscopic imaging to dry, dead samples.
A research team from Purdue University, USA, has now gotten around these limitations for visualizing live cells, with a mid-IR photothermal (MIP) imaging approach that combines the chemical sensitivity of a QCL with the fine spatial resolution of a visible-light laser (Sci. Adv., doi: 10.1126/sciadv.1600521). The team’s setup has allowed the researchers to achieve chemical images of structures less than a micron across, in live worms and other biological samples.
Thermal lensing
Conventional mid-IR absorption spectroscopy images an object by measuring spatial variations in its chemical composition, based on the absorption frequencies of particular molecular bonds. Modern QCLs are especially good with this work, as they can pinpoint frequencies tuned to specific molecular vibrational bands, and dramatically speed up the process of building a chemical image of the sample. The strong absorption of mid-IR wavelengths by water, however, tends to swamp the signal from other chemical species, making the technique all but useless for living biological samples.
To get around that roadblock, the Purdue team—led by OSA Life Member Ji-Xin Cheng at the Weldon School of Biomedical Engineering—took advantage of a side-effect of mid-IR absorption: the heating of the sample. The temperature increase with infrared absorption locally changes the refractive index of the absorbing medium, creating a sort of “thermal lens.”
That lens effect is similar, according to the study’s first author, postdoctoral research associate Delong Zhang, to “the mirage effect that you see on a hot summer day over a road surface.” And, even better, while thermal lensing can be a pronounced effect for a variety of biomolecules, the effect of temperature changes on water’s refractive index (at least near room temperature) is relatively weak. That offered the prospect of using thermal lensing as a proxy measure in mid-IR imaging of live cells, and thereby circumventing the signal-sapping disadvantages of the watery background.
Two lasers
To use this MIP imaging for ultra-fine cellular pictures, the Purdue team relied on an ingenious setup involving two lasers: a mid-IR pulsed QCL, tunable between 1345 and 1905 cm–1, to provide the thermal-lensing signal; and a 785-nm continuous-wave probe laser, to read that signal at high resolution.
In the setup, the two beams are collimated with a silicon dichroic mirror, and the combined beam is pushed up through the sample and into an objective lens. The mid-IR QCL pulses cause the target area of the sample to heat up, leading to local refractive-index changes.
Those changes, in turn, form a local thermal lens that causes a deviation of the visible-light probe laser photons, with the amount of the deviation dependent on the specific chemistry of the target. The spatial distribution of the visible-light probe photons is then read at a photodiode, and their behavior is computationally tied to the pulse frequency of the QCL to pull out the photothermal signal.
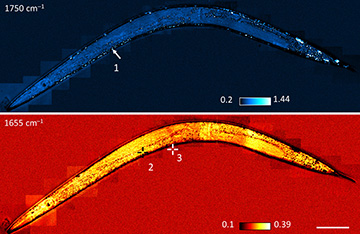
MIP imaging allowed the Purdue team to visualize tiny, sub-micrometer lipid droplets (dots) in C. elegans worms—and even to acquire pinpoint spectra of the droplets to infer their chemical composition. (Scale bar: 200 μm) [Image: From Zhang et. al., Sci. Adv., doi: 10.1126/sciadv.1600521. This work is licensed under CC BY-NC.] [Enlarge image]
Lipid droplets
The result of the process, repeated in scans across the sample, is a surprisingly high-resolution view of the internal structure of cells and organisms. The Purdue team reports that it can map 10-micromolar variations in chemical concentration, on a spatial scale of less than 1 μm. The researchers could demonstrate the imaging in action by training their collimated beam on live C. elegans worms; in that test, they managed to image tiny, sub-micron lipid droplets in the worms, and even to obtain photothermal spectra of individual droplets to verify their composition.
The Purdue researchers are now working to patent the label-free technique, which they believe could provide insights into drug delivery and disease processes. One important hurdle to clear toward commercial operation, and a key next step for the team, will be increasing the speed of the process—which currently takes eight seconds per image—to a rate that can capture moving cells and molecules in dynamic, real-world environments.