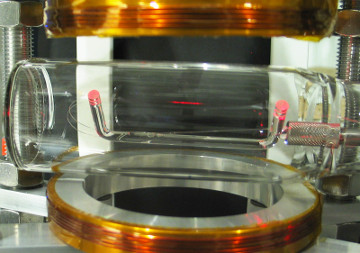
In the nanofiber portion, strong coherent Bragg scattering off of a trapped linear ensemble of around 1,000 atoms causes a reflection of a significant fraction of light (bright red section) launched into the fiber. [Image: J. Appel/Univ. of Copenhagen] [Enlarge image]
Mirrors are among the most fundamental of optical components, at virtually all length scales. Now, two research groups, one based in France and one in Denmark, have independently demonstrated an approach to making mirrors that are very small indeed, consisting of one to two thousand atoms (Phys. Rev. Lett., doi: 10.1103/PhysRevLett.117.133603 and 10.1103/PhysRevLett.117.133604).
The research groups believe that these 1-D mirrors—which consist of lines of atoms, optically trapped in the evanescent field outside of tapered nanofibers—could prove useful as optical switches or single-photon coupling components in photonic circuits, and as a platform for experiments in quantum information.
Bragg reflection
The reflection in question is Bragg reflection—the coherent scattering of light from individual, regularly spaced particles. The concept has its roots in X-ray crystallography. For a crystal lattice consisting of multiple planes of atoms, the so-called Bragg condition holds that if the path difference that must be traveled for light hitting two different crystal planes amounts to an integer multiple of the light’s wavelength, the two waves will interfere constructively.
That relationship is summed up in the famous equation 2d sin θ = nλ, where d is the space between lattice planes, θ is the light’s scattering angle, and n is an integer. And, as the equation shows, for incident light perpendicular to the crystal planes, the system will behave as a mirror if the spacing between the planes is exactly half the wavelength of the light.
Tapered nanofibers and evanescent fields
The two European groups—one led by Julien Laurat at the Pierre and Marie Curie University in Paris, and the other by Jürgen Appel of the University of Copenhagen—realized that a 1-D Bragg mirror could be created from strings of atoms, if they could be arrayed and held in a line at precisely the right spacing. But how to do so? Both groups found the answer in systems involving tapered nanofibers. These systems consist of conventional silica fiber in which a portion has been tapered down to a width of a few hundred nanometers—below the wavelength of the light sent through the fiber.
Because of that geometry, as light passes through the fiber and into the subwavelength region, a portion of its energy is squeezed out into an “evanescent field” surrounding the nanofiber section. Further, that evanescent field can be tuned into a “two-color” standing-wave trap using the right combination of red and blue light, guided through the fiber. As has been demonstrated in the past several years, laser-cooled atoms can be slowed down and captured in the nodes in that standing wave, offering the opportunity for coupled light-matter systems that can be used to create applications such as fiber-based optical memory.
Making the mirrors
For their 1-D Bragg mirrors, the two European groups were able to create precisely spaced lattices (using red or infrared light) consisting of only a comparatively tiny number of atoms—around 1,000 for the Appel group and 2,000 for the team led by Laurat. They then sent a beam of probe light through the fiber, and found that the trapped atoms Bragg-reflected a significant portion of the probe beam. For the Appel team, the 1-D grating reflected nearly 12 percent of the beam—“more light than a solid slab of glass,” according to the paper. And the Laurat group was able to achieve guided-mode reflectances of a whopping 75 percent, owing in part to the larger number of atoms involved.
Both groups note that, in addition to their interest as mirrors, the systems are intriguing because their reflectance can be modified or switched into a nonreflecting state by modifying the pump light used to create the lattice. And, the Appel group notes in its paper, integrating components such as an optical cavity within the fiber could in principle allow for single-atom reflectivity of “near unity,” opening up the prospect of strong single-photon effects in the system. That, in turn, could make these systems “an attractive playground for experiments” in quantum information.