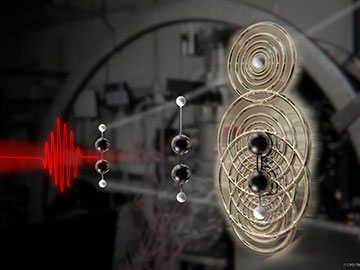
In the laser-induced electron diffraction (LIED) scheme, an intense laser kicks off an ionization reaction that strips off an electron and causes a hydrogen ion (white ball) to dissociate from the parent molecule. After 9 fs, the laser drives the ionized electron back to scatter off of the dissociating molecule. The electron-scattering data are used to create snapshot of the molecule in the act of breaking up. [Image: ICFO–The Institute of Photonic Sciences and Scixel]
Researchers who want to capture snapshots of chemical reactions in the act have generally faced a difficult tradeoff between resolution in time and resolution in space. Now, in a tour de force of ultrafast imaging, an international team of scientists reports that it has overcome that tradeoff, and has taken snapshots of a molecular reaction with a temporal resolution of 0.6 femtoseconds and a spatial resolution as small as 0.05 Ångstroms—less than the width of an atom (Science, doi: 10.1126/science.aah3429).
The team achieved the feat by using laser fields to orient a hydrocarbon molecule and push it into an ionization reaction, and then send the molecule’s own ionized electrons back to scatter off of the molecule and obtain information on its evolving structure. The result is a sort of “molecular selfie”: a real-time snapshot of a molecular reaction using electrons from the molecule itself.
Bridging the spatial-temporal gap
Imaging chemical reactions requires a temporal resolution of a few femtoseconds and a spatial resolution at the picometer level. And that’s a bit of a quandary. Pulsed-laser and X-ray techniques can offer the requisite time resolution, but only at a relatively coarse spatial resolution of a few Ångstroms, or hundreds of picometers. Electron scattering offers a much tighter, few-picometer spatial resolution, but only at time resolutions in the hundreds of femtoseconds.
To resolve that quandary, the research team—led by OSA Fellow Jens Biegert of ICFO–The Institute of Photonic Sciences in Barcelona, Spain, and Chii-Dong Lin of the Kansas State University, USA, along with researchers from other institutions in the Netherlands, Germany, and Denmark—turned to a technique that combines the best of both approaches: laser-induced electron diffraction (LIED). In LIED, an ultrafast laser ionizes an electron from the molecule of interest, and then accelerates the electron back to the molecule, to be rescattered and collected. A second tool, called a reaction microscope—a kind of momentum spectrometer that captures the vector paths and energies of the scattered electrons—is then used to infer the structure of the scatterer.
Two laser fields
LIED is actually not a new idea; it was first proposed 20 years ago by Paul Corkum as a possible window into ultrafast molecular dynamics. Reaction microscopes, too, have a long pedigree in atomic, molecular and particle physics. But it was only last year (Nat. Commun., doi: 10.1038/ncomms8262) that the Biegert–Lin team suggested the specific setup that has enabled the high-resolution snapshots in the current study.
That setup begins with an ICFO-built optical parametric chirped-pulse-amplification (OPCPA) laser, tuned to produce simultaneous outputs at two infrared wavelengths: a 1700-nm pump pulse and a 3100-nm main pulse. The two laser fields are trained on a jet of acetylene (C2H2) molecules passing through the reaction microscope apparatus. The team chose acetylene for the experiments because it is a well-studied molecule, with a number of potentially interesting, extremely rapid reaction pathways to study.
As an acetylene molecule falls through the apparatus, the 1700-nm pump field acts as a control field, aligning the molecule in space. The 3100-nm pulse then ionizes the molecule, triggering a dissociation reaction, in which a proton (H+) separates from the parent C2H22+ ion, leaving an ethynyl ion (C2H+). The ionized electrons re-accelerate back to the dissociating molecule and scatter off of it, and the detailed angular-momentum distributions of the scattering are read in the reaction microscope. The team then uses computational modeling to translate those distributions to structural information on the molecule, and in turn uses that information to build snapshots of the molecule as it breaks apart.
“Mind boggling” length and time scales
The whole process, from ionizing the electron to sending it back to obtain structural information, takes a mere nine femtoseconds. And by leveraging, in the post-collection analysis, some additional knowledge of time-energy correlations for the ionized electrons, the team was able to obtain its molecular snapshots with an estimated temporal resolution of 0.6 fs—some ten times shorter than the optical cycle of the 3100-nm laser field. Further, because of their ability to orient the molecule using the 1700-nm control field, the team could show how the molecule’s dynamics differed depending on its orientation with respect to the reaction-triggering laser.
Team leader Biegert summed up the accomplishment this way: “We took one electron, steered it along a specific path with the laser and scattered it off an isolated molecule to record its diffraction pattern”—achieving a molecular view on length and time scales that he describes as “mind-boggling.” Having proved the concept out with acetylene, the team is, he says, “eager” to try the technique out on other molecular species, such as specific catalysts and biomolecules.